Table of Contents
Fossil fuels have been used by people for energy for the past two hundred years. They are a convenient, energy-dense substance that contains hundreds of millions of years of photosynthesis. But there is a limited quantity, and using fossil fuels has a hugely negative effect on the climate of the Earth.
Without natural photosynthesis, we would not be here. It made the oxygen we breathe on Earth and it makes the food we eat, But it will never be efficient enough to supply fuel for us to drive cars, so we will need something else.
The most significant difficulty that many people are unaware of is that nature has no solution for the amount of energy we consume. We will have to outperform nature, which is terrifying.
Scientists are looking on “artificial photosynthesis”—reworking a plant’s system to produce our own fuels. However, the chemical machinery in a single leaf is extremely complex and difficult to apply to our own goals.
What is Artificial Photosynthesis?
The new novel system for artificial photosynthesis outperforms earlier artificial systems by an order of magnitude. Artificial photosynthesis, as opposed to regular photosynthesis, which produces carbohydrates from carbon dioxide and water, has the potential to produce ethanol, methane, and other fuels.
Photosynthesis in nature is carried out by a number of highly complicated protein and pigment complexes. They absorb water and carbon dioxide, disassemble the molecules, and rearrange the atoms to form carbohydrates—a lengthy chain of hydrogen-oxygen-carbon compounds. However, scientists must modify the interactions to obtain a new arrangement with only hydrogen surrounding a juicy carbon core—CH4, generally known as methane.
In contrast with a regular mode of photosynthesis, which deals with the production of glucose for the growth of plants, artificial photosynthesis aims at the generation of clean fuels like hydrogen and methanol that can be used in automobiles and industries without the emission of any undesirable effluents.
In its essence, the artificial photosynthesis involves collecting sunlight through specialized materials and catalytic systems to disassociate water content and create energy-dense molecules. This method not only utilizes renewable energy resources but also could incorporate and transform carbon dioxide enabling the solution of two prominent worldwide problems: the shortage of clean power and the excessive carbon emissions.
Artificial photosynthesis provides another avenue in the quest for sustainable energy supplies if and when fossil fuel resources are depleted and as concerns over global climate change increase. Most renewable resources, like solar and wind, are quite valuable but do suffer from issues of energy storage and intermittency. The one key advantage of artificial photosynthesis is that, within this process, chemical energy is produced that can be stored and transported easily as fuel.
This has realized materials science breakthroughs such as specific catalysts and semiconductors that efficiently capture sunlight and drive the needed chemical reactions. Further strides by scientists in this area could make artificial photosynthesis a prime driver toward a carbon-neutral future that would mean a way into energy security as well as environmental sustainability.
The Inspiration: How Natural Photosynthesis Works
The process of turning sunlight—more especially, carbon dioxide and water—into food is photosynthesis. Most of the chemical energy moving through the biosphere comes from photosynthesizing plants, algae, and microbes. Most of the biomass they generated also resulted in the fossil fuels driving most of our current society. On land, in the sea, and in freshwater habitats photosynthesis occurs.
Evolution of first photosynthesizing single-celled bacteria occurred about 3.5 billion years ago. About a billion years later, the ensuing growth in atmospheric oxygen—a consequence of photosynthesis—was crucial in determining the course of life on Earth during the last 2.5 billion years. The great majority of land, freshwater, and sea life today depends on oxygen for breathing—the metabolic process that converts food into energy.
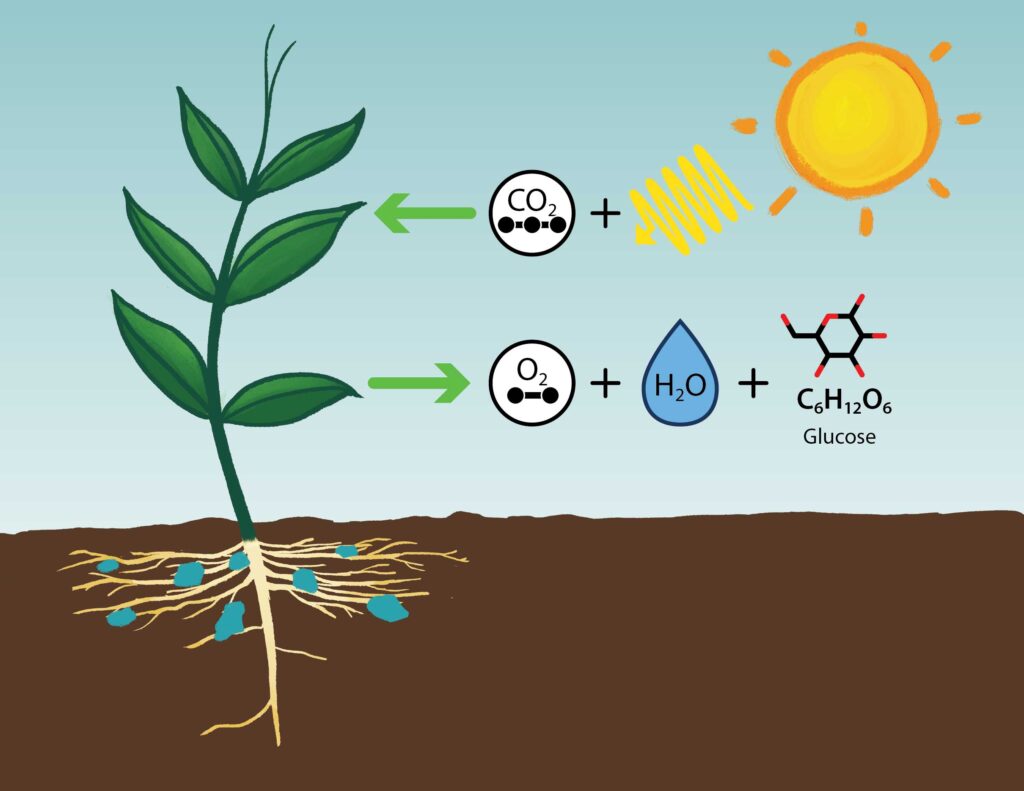
Different environmental circumstances influence the rate of photosynthesis in ecosystems by means of:
- Conditions of the climate, like the amount of sunlight at different regions, temperature, and rainfall For instance, ecosystems near the poles have lower production and biomass than ecosystems near the equator. This is because ecosystems near the equator get more sunlight and rain than ecosystems near the equator.
- Nutrients, especially nitrogen and phosphorus, can lower output when they are scarce but raise it when they are plentiful. Photosynthesizing creatures take nutrients from their surroundings and put them back into the soil when they break down.
- Abiotic natural factors include soil quality (often linked to nutrient levels), wildfires, the acidity of the water, and the amount of oxygen in the air.
- Species interactions, such as the things that different species do to help each other and the ways that they fight for things like space, light, and water.
- Species that make other species less or more successful change the sizes of their populations, which in turn changes output and biomass.
People have changed the rate of photosynthesis and, by extension, the output of ecosystems by doing many things, such as:
- Loss of habitat, cutting down trees, and building cities are all things that damage ecosystems and take plants and trees from them.
- Activities in agriculture that make more food available to feed the world’s growing population.
- The use of fertilizers in farming that raise the levels of nutrients in the earth or water, mostly nitrogen and phosphorous.
- People use freshwater, which can reduce the amount that plants and trees in an environment can use.
- Letting out waste and pollutants, which can stop plants from growing and reproducing or even kill them.
- Burning fossil fuels, farming, and cutting down trees are all examples of things that release carbon dioxide and other greenhouse gases that warm the planet. Some plants may be able to do more photosynthesis when the carbon dioxide level goes up, but this can also make plants less healthy.
- Bringing in invasive species that fight with native plant or algae species for food, water, light, or other resources, which lowers the numbers of native species.
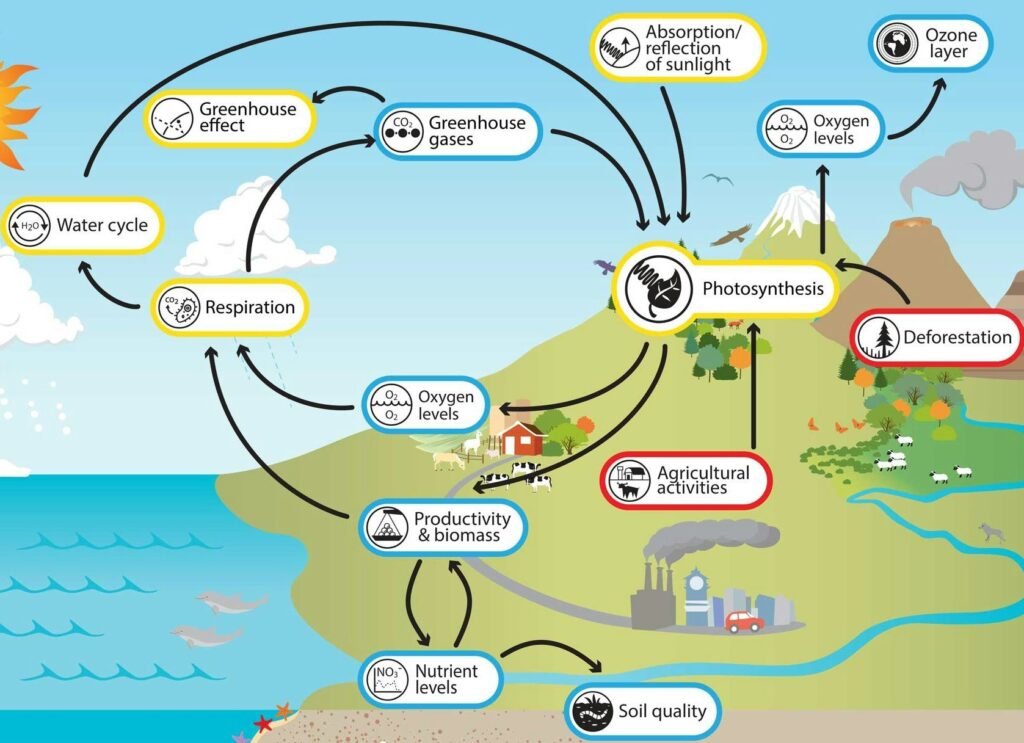
Why Artificial Photosynthesis? The Need for Clean Energy Solutions
Every thirty minutes, the sun generates enough energy on Earth’s surface to meet humanity’s energy needs for a full year. Unlike fossil resources, which are unevenly distributed over the planet, producing political unrest or availability concerns, sunshine is abundant and geographically spread. Many say that solar energy is the only source capable of powering society in the long run. In other terms, the sun represents our only hope.
Natural photosynthesis is exclusively responsible for all of the energy burned around the earth. All of the energy circulated throughout the biological community originates from photosynthesis, which occurs in the organisms at the bottom of the food chain. Despite this, photosynthesis is also responsible for the energy stored in fossil fuels like coal and petroleum. With this in mind, it makes theoretical sense to attempt to extract useable energy directly from a biomimetic approach to this natural process.
The notion of artificial photosynthesis is similar to that of solar panels, which can be found on roofs or in fields. However, photovoltaic cells used in solar panels are intended to capture solar energy and convert it into electricity for immediate use. While this can be beneficial, the electricity generated by solar panels is restricted by its reliance on weather and time, which is further constrained by the fact that it cannot be stored adequately by batteries at this time.
In contrast, while artificial photosynthesis systems need on sunlight, semiconductors can collect solar energy and store it in the chemical bonds of fuel for later use. In this method, energy is stored densely and cheaply in the form of fuel, as opposed to the costly and unsustainable option of battery storage.
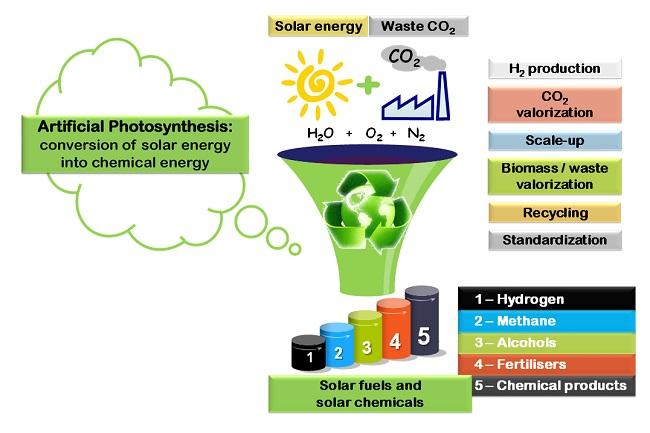
How Artificial Photosynthesis Works: Key Concepts and Process
Every year, plants convert 1,102 billion tons (1,000 billion metric tons) of CO2 into organic matter, i.e., food for animals, using just sunlight as an energy input. And that’s just using 3% of the sunlight that reaches Earth.
In this article, we’ll look at artificial photosynthesis and see how far it’s come. We’ll find out what the system has to be able to do, check out some current methods of achieving artificial photosynthesis and see why it’s not as easy to design as some other energy-conversion systems.
To replicate the photosynthesis that plants have accomplished, an energy conversion system must be able to do two critical tasks. Harvest sunlight and divide water molecules.
Plants execute these activities with chlorophyll, which absorbs sunlight, and a set of proteins and enzymes that use that sunlight to degrade H2O molecules into hydrogen, electrons, and oxygen. The electrons and hydrogen are then employed to convert CO2 into carbohydrates, while oxygen is released.
For an artificial system to function for human requirements, its output must alter.
Artificial Photosynthesis utilises solar energy to generate hydrogen from water. Utilising hydrogen and CO₂ released from industrial facilities and power stations to synthesise olefin will significantly transition from CO₂-emitting chemical production methods to a CO₂ absorption methodology.
Three processes in Artificial Photosynthesis
- Splitting water into oxygen and hydrogen using sunlight and a photocatalyst
- Using a separation membrane to split hydrogen from the emitted mixed gas, consisting of hydrogen and oxygen
- Using catalyst to create a reaction between hydrogen and CO₂ to produce olefin
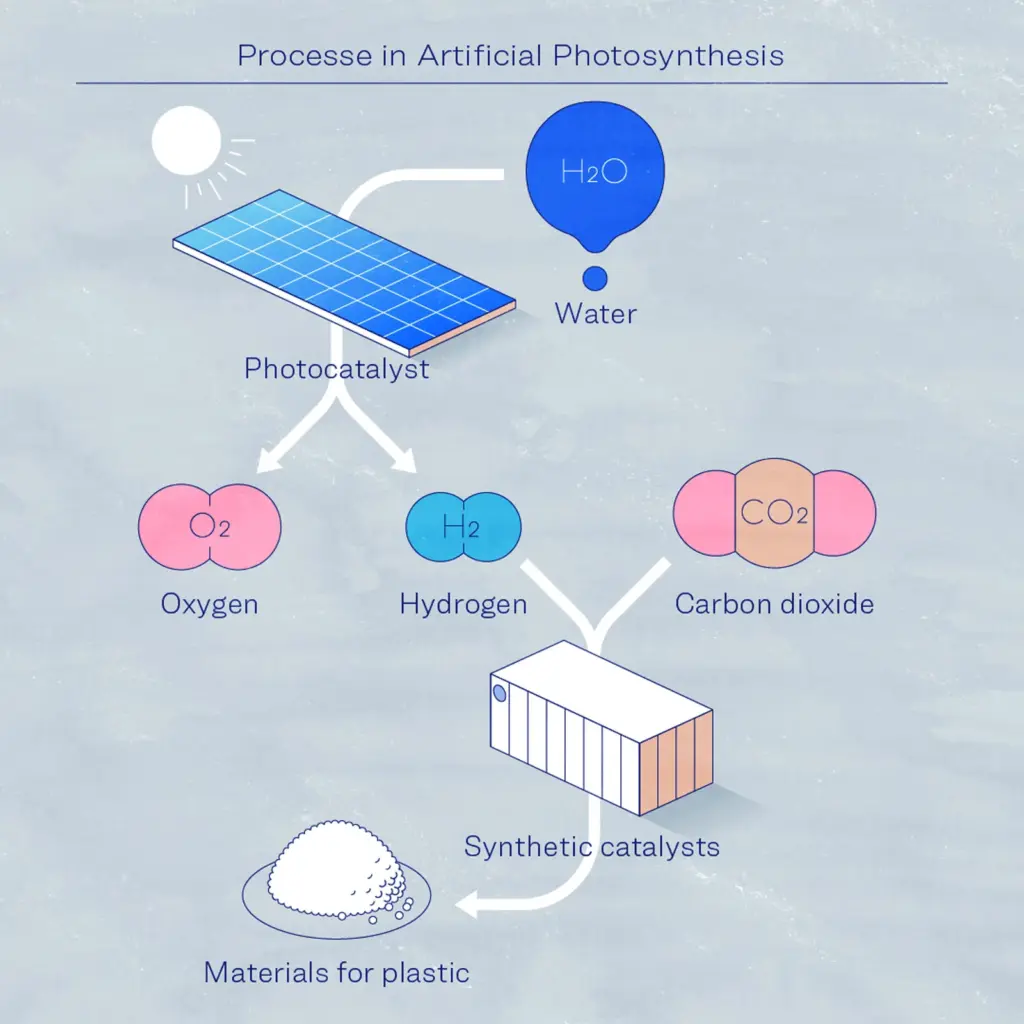
Splitting water into oxygen and hydrogen using sunlight and a photocatalyst
The photocatalyst plays a vital role in the conversion of water into hydrogen and oxygen. Light is shone on the catalyst, which is in sheet shape and saturated in water, and it separates the water into hydrogen and oxygen without the use of electricity. This process uses solar energy, which eliminates CO₂ emissions during manufacture. An important component in Artificial Photosynthesis is the energy conversion efficiency rate, which refers to how efficiently energy can be converted while creating hydrogen from solar radiation.
Using a separation membrane to split hydrogen from the emitted mixed gas, consisting of hydrogen and oxygen
The hydrogen-oxygen gas mixture is explosive, thus it is critical to separate the hydrogen and oxygen safely and efficiently. In this project, in addition to developing a high-performance separation membrane (for separating hydrogen from mixed gases), an exceedingly safe separation module is also being developed.
Using catalyst to create a reaction between hydrogen and CO₂ to produce olefin
A synthetic catalyst is essential for the creation of olefin. The synthesis process involves reacting the separated hydrogen with CO₂. A catalyst and process technologies for high yields and high-level production were developed, and proof-of-concept testing has already been successful on a small pilot scale. This sequence of procedures produces olefin, which is then used to make plastic.
Comparison between natural and artificial photosynthesis.
Natural Photosynthesis | Artificial Photosynthesis | |
Energy Source | Sunlight | Sunlight |
Reaction Center | Chlorophyll in photosystem | Photo-electrochemical cells |
Energy Storage | Glucose (a carbohydrate) | Hydrogen or other solar fuels |
Oxygen Evolution | Yes, from water | Yes, from water |
Carbon Fixation | Yes, carbon dioxide into glucose | Potentially, carbon dioxide into carbon-based fuels |
Efficiency | 3–6% | Variable, still under development |
Product Utility | Mainly food and biomass | Mainly fuels for energy and industry |
Environmental Impact | No negative impact, reduces CO2 | No negative impact, could reduce CO2 |
Catalysts | Enzymes | Man-made catalysts |
Rate of Reaction | Relatively slow because of enzymatic constraints | Potentially faster with optimized catalysts |
Operating Conditions | Ambient temperature and pressure | Variable, can be optimized for reaction |
Evolution and Optimization | Billions of years of natural selection | Still under development, ongoing optimization |
Dependence on Water | High, water is electron donor | High, water often used for proton/electron source |
Lifetime/Durability | Limited by organism’s lifespan | Potentially long, dependent on material degradation |
Challenges and Limitations: What’s Holding the Technology Back?
Natural photosynthesis has a high quantum efficiency, which leads to effective charge separation, but the overall conversion of solar energy to chemical energy is rather poor, at around 1%. Artificial photosynthesis demonstrates efficiency of up to 10% or greater. One of the most important hurdles in artificial photosynthesis is developing a cost-effective, efficient, and stable catalyst material.
The organic-based catalyst tends to lose stability after many uses, corroding or blocking the operation of system equipment. Many metal-based catalysts have been tested, and the hunt for cost-effective and stable alternatives has been ongoing for at least ten years. Simulating a complex process such as photosynthesis is extremely difficult.
Another significant obstacle in imitating a natural process is the complicated molecular architecture present in photosynthetic organisms. Researchers are having difficulty recreating the level of complexity that it entails. Many catalysts have been synthesised over the last few decades; unfortunately, many are unstable.
Nonetheless, using supramolecular methods and nanotechnology, scientists can readily modify the workings of their gadgets via structural and molecular compositions. Studies on molecular catalyst heterogeneity are limited since it is difficult to replicate the intricacies found in natural photosynthesis. The development of effective molecular catalysts will allow the field of APS to advance into a viable system.
The Future Potential: Artificial Photosynthesis in a Sustainable World
To help people around the world get through this tough time, experts are working hard to find sources of clean energy that can be used. The aeroplane was based on the way birds fly. In the same way, natural photosynthesis can be used to copy the functions of photoautotroph organisms that can survive on their own, which will hopefully one day lead to a world that can survive on its own.
Artificial photosynthesis is already working well and is simpler, better at moving charges, and better at absorbing light across a wider range of wavelengths than wild catalytic systems. In the same way that solar panels can be put on roofs to provide an extra source of electricity, future artificial photosynthesis devices will be able to power houses by storing energy for later use.
More than 60% of the world’s oil supply is lost through transportation. Electric cars are a great option, but new models of cars that run on hydrogen, which is made when artificial photosynthesis is done, were said to change the whole car business. These hydrogen-powered cars only need a short time to refuel and are good for the earth.
Natural photosynthesis provides all of the energy needed to life on this planet. In addition, photosynthesis gives energy to fossil fuels. It took billions of years for protobionts to evolve into multicellular photosynthetic systems. To duplicate natural photosynthesis, it may require more than a decade of significant research before artificial photosynthesis is ready for industrial use.
As a result, attempting to capture energy from this natural process via a biomimetic technique is critical. The effort to develop a self-sustaining system employing artificial photosynthesis is still in its infancy. Many viable variants of artificial photosynthesis have been developed, although not all models are perfect and have drawbacks such as efficiency, instability, and financial costs. The adoption of AI in photosynthetic research is still in an underdeveloped stage in many countries.
The search for a cost-effective, resilient, and scalable artificial photosynthesis is ongoing in organisations such as the Liquid Sunlight Alliance (LiSA) and the Centre for Hybrid Approaches in Solar Energy to Liquid Fuels (CHASE). The numerous attempts to actually apply artificial photosynthesis fall short of several efficiencies, although solar fuel production via natural photosynthesis is still possible in the laboratory.
The scientific community is well-versed in the words and working principles of solar fuel, artificial leaves, and artificial photosynthesis, and they are working hard to deliver energy using clean, green alternatives around the world.